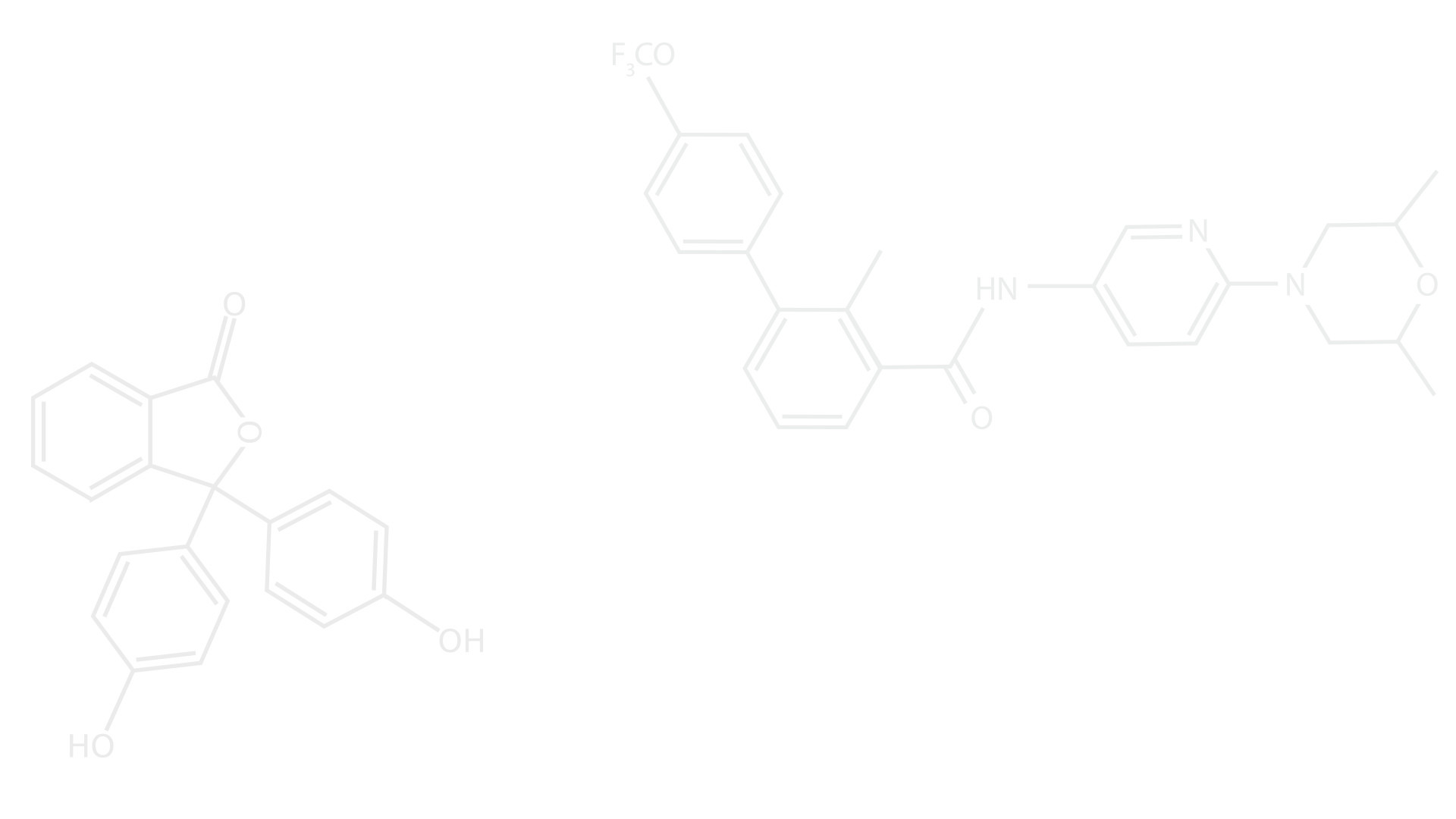
Story of the quarks
​
Inward Bound Journey
​
​
​
​
​
​
​
​
The inward bound journey that took us deeper and deeper into matter has culminated at quarks at the end of the twentieth century. At the
beginning of the twentieth century we started with the atom of size , went through the nucleus of size , nucleon (proton
or neutron) of size and finally quarks of size smaller than . This journey has taken a 100 years.
​
The old story (Strong interactions and hadrons)
Particles having strong interactions such as protons and neutrons are called hadrons. Their story is very complicated. I have simplified it a little and presented it here.
​
After the discovery of the atomic nucleus in 1911 by Rutherford, proton was recognized to be the nucleus of the Hydrogen atom. Then in 1932, Chadwick discovered the neutron. After that, nuclei were understood as made of protons and neutrons and it was found that they are bound inside the nuclei because of the strong interactions among them with a short range
​
In the year 1934, Yukawa discovered that this short-ranged strong interaction is caused by the exchange of a particle of about 100 MeV. Around that time a particle of roughly that mass was discovered in cosmic rays.
​
Confusion prevailed for many years. Finally it became clear that Yukawa's particle and the cosmic ray particle are different. The former is called pion or pi meson and the latter is called muon. It is the experimental discovery of pion decaying into muon by Powel and Occialini that
clarified the confusion and this happened only after 1947.
​
An important milestone was the discovery of SU(2) symmetry by Heisenberg in 1935. The strong force between proton and proton, neutron and neutron as well as proton and proton were found to be equal. Their masses were about the same (1940 Mev). These facts induced Heisenberg to propose that this symmetry between proton and neutron is a result of SU(2) symmetry. This symmetry of two objects is the first internal symmetry that later led to more internal symmetries in elementary particle research.
​
Quantum Field Theory was an essential ingredient in our understanding of elementary particles and the forces between them. In fact Yukawa had used quantum field theory in making his prediction of pion as the carrier of the strong force. However the quantum field theory of strong interactions did not progress far, because nobody knew how to calculate using a theory where the interaction was strong.
​
In 1954 Enrico Fermi and Herbert Anderson discovered using the Chicago cyclotron, a new hadron called Delta of mass 1238 MeV. This hadron could decay into a nucleon and a pion by strong interactions since its mass is higher than the sum of masses of the nucleon and pion. So the hadron manifests itself as a resonance in nucleon-pion scattering. Delta was the first resonance hadron to be discovered.
Important milestones in the history of strong interactions
​
Scientists and their discoveries
​
​
​
The years 1957 to 1965 were the golden age of hadrons. Hundreds of hadrons were discovered. As a result of this deluge, quantum field theory was given up. Under the leadership of Geoffrey Chew, a new philosophy called S-Matrix theory was developed. As a result, many new concepts such as dispersion relations, nuclear democracy, Regge poles, bootstrap were studied. However ultimately this route turned out to be a dead-end.
​
Another route spearheaded by Murray Gell-Mann became successful. It started with SU(3) symmetry which is a symmetry of three objects and led to the discovery of quarks.
​
Many new hadrons such as K mesons, Lambda, Sigma and Xi were discovered in cosmic rays in the 1950's. They were produced copiously, but decayed only weakly. This was a puzzle that was solved by Gell-Mann and Nishijima by the invention of a new property or quantum number called strangeness. The new hadrons have this property while the older hadrons such as proton and neutron are non-strange.
​
Including this new quantum number, SU(2) symmetry can be enlarged to SU(3). Sakata and his group proposed this as the symmetry of three particles proton, neutron and Lambda. This was called Sakata model.
​
​
​
​
​
​
But a question arose. Although proton, neutron and Lambda do not belong to the SU(3) triplet, SU(3) symmetry needs a triplet. Only then, the 3 in SU(3) will have meaning. So where is the triplet?
​
At this point I must describe something that happened In Bangalore in 1961.
​
The Bangalore incident
The first Summer School of the Tata Institute of Fundamental Research, Bombay was held at the Institute of Science, Bangalore in August 1961.
​
Murray Gell-Mann and Richard Dalitz gave the lectures. Apart from research students like me, Professors like Homi Bhabha, MGK Menon, Yash Pal and SN Biswas were also in the audience.
Gell-Mann lectured about his recently developed Eightfold Way. His paper had not been published. So it was fresh from the anvil.
​
Dalitz asked him about the triplet. Why did he leave the triplet which was necessary to give meaning to SU(3)? Although Dalitz persisted with his question repeatedly, Gell-Mann escaped without answering it.
​
If Gell-Mann had answered Dalitz's question, quarks would have been born in Bangalore in 1961. That did not happen. Quarks had to wait until 1964. If any of us had answered it, it would have been a great Indian discovery. This was an important missed opportunity in the history of Indian Science.
​
Quarks
Gell-Mann and Zweig proposed the quarks independently in 1964. But it took a few more years for the quarks as real particles to be recognized. Gell-Mann himself regarded them as mathematical objects that are useful for understanding hadrons.
The three quarks are called u,d and s. Therefore in Sakata's SU(3) we must take u,d and s as the triplet instead of proton, neutron and Lambda. All the hundreds of hadrons are made of these quarks.
​
But most physicists refused to believe in quarks. An important reason for that was the S-Matrix philosophy which was dominant at that time. The idea that some of the strongly interacting particles such as quarks were more fundamental than others was repugnant to nuclear democracy. In 1968, Chew, in a lecture at TIFR, Bombay claimed that he can prove by using relativity and quantum theory that there can be no particle more fundamental than proton, neutron and other hadrons.
​
In this prevalent quark-phobia atmosphere, there were a few bold souls that took the idea of quarks as constituents of hadrons seriously and started exploring the consequences. They were Dalitz, AN Mitra and Morpurgo. In particular, maybe because of the Bangalore incident, Dalitz began to work out the the consequences in detail. In the International conference on High Energy Physics held in Berkeley in 1965(?), he showed how all the properties of hadrons agree with the assumption that they are made of quarks. This surprised everybody and became a turning point in the history of quarks.
​
But physicists started believing in quarks only after two more experiments. One was the experiment of high energy scattering of electrons on proton at Stanford, California by Friedman, Kendal and Taylor in the late 60's. The other was the revolutionary discovery of the psi particle in 1974.
​
Finally quarks triumphed. There is a parallel between the story of quarks and the story of atoms. Famous scientists like Ernest Mach and Ostwald did not believe in atoms. Boltzman was the chief protagonist on the side of atoms. He waged a heroic struggle and ended up committing suicide. Finally, only after Jean Perrin proved the correctness of Einstein's formula for Brownian motion based on atoms, that atoms won.
​
In the Stanford experiment, high energy electrons were made to collide on protons. The energy of the hadrons was converted into many hadrons. Analysis of the results of these experiments showed that there are point-like particles inside the proton and they were found to be quarks.
​
This experiment was somewhat similar to Rutherford's 1911 experiment when he deduced the existence of point-like nucleus inside the atom by colliding alpha particles against gold atoms.
​
Let us now come to the 1974 the psi particle revolution. The peculiarity of psi was that it decayed very very slowly and so its lifetime was 10,000 times longer than expected. This was like the discovery of some tribe of humans in a forest living for a million years! This led to the discovery of a fourth quark, the c quark. Psi particle was made of a c and an anti-c (usually denoted as cbar).
​
From these two experiments, electron scattering and psi discovery, an important property of the strong interaction among quarks was discovered. It is called Asymptotic Freedom. When energy becomes very large, quarks behave like free particles.
​
It was soon discovered by Gross, Wilczek and Politzer that only Nonabelian Gauge theory (also called Yang-Mills theory) has this property of Asymptotic Freedom. Soon after, Gell-Mann, Fritzsch and Leutwyler proposed the Nonabelian gauge theory based on the color quantum number of quarks (that was already known), called Quantum Chromodynamics (QCD) as the correct theory for strong interactions. This was the birth of QCD. All this happened in 1972-73.
​
In QCD, quarks interact through the exchange of gluons, just as, in QED (quantum electrodynamics) electrons interact through the exchange of photons. They are called gluons since they glue the quarks to make the hadrons.
​
In the history of strong interactions, Gell-Mann's role was very big. Strangeness, SU(3), quarks, QCD - in all these he is there.
​
Gell-Mann had a knack for coining names. Strangeness, quarks, gluons, color, QCD - all these are names coined by him.
​
​
​
​
​
​
​
​
​
​
​
​
​
​
​
​
​
New quarks
​
​
​
​
​
So far we have 6 quarks in total:
u c t
d s b
​
These are called three generations of quarks.
​
Similarly there are three generations of leptons (particles that do not have strong interactions):
​
​
​
​
​
​
Confinement of quarks
An important question arises. If we collide one atom on another atom, the electrons will come out. In the same way, if we collide a proton against
another proton, will the quarks come out? No. Why?
​
The answer is buried in QCD. Just as QCD has asymptotic freedom, it has infrared slavery also. As a consequence quarks are confined within the
hadrons. Confinement is an important characteristic of QCD.
​
​
​
​
​
​
​
​
Therefore, if we try to increase the distance between two quarks, the attractive force between them goes on increasing and it would be impossible to separate the quarks. Even very high energy collisions between protons will not liberate the quarks from their confinement.
​
Asymptotic freedom of QCD has been proved by mathematics. But confinement has not been proved. This remains as a challenge in Physics and Mathematics.
​
World's brightest theoretical physicists and fastest computers have been trying to prove confinement in QCD. But no success so far. In the year 2000, CLAY Institute in Canada has announced the proof of confinement as one of the Millennium problems in Mathematics. A million dollars will be awarded to one who proves it.
​
Reference
"From Atoms to Quarks and Beyond: A Historical Panorama" G Rajasekaran, in India in the World of Physics, Then and Now (History of Science, VolXIII, Part I) Ed: AN Mitra, Pearson Longman, p361 (2009), Also in ArXiv:Physic/0602131.
Atom
10
-8
cm
Nucleus
10
-12
cm
Nucleon
10
-13
cm
quark
10
-17
cm
?
10
-17
cm
10
-13
cm
10
-8
cm
10
-12
cm
10
-13
cm